Definitely among the most powerful stellar explosions in the Universe, Type Ia supernovae are actual Earth-size space thermonuclear-bombs, able to give us precious information from the most far away galaxies.
What is dark energy? How were the heavy elements from iron to uranium made? How exactly does binarity affect the life of stars? What is the ultimate fate of the Universe? These questions have two aspects in common: 1) they are all amongst the greatest unanswered questions in physics; 2) they all crucially depends on our knowledge of Type Ia Supernovae: the thermonuclear explosion of hot, dense objects, called “white dwarfs”, in stellar systems. Yes, people usually associate the word “supernova” to the death of massive stars running out of fuel… this is not always true.
Despite the importance of Type Ia supernovae, it is now known that the nature of these objects may be more complex than once thought, beginning with their origins. Do Type Ia supernovae arise from white dwarfs accreting matter from a younger companion? Or do they merge with another white dwarf? Astronomers looked for the signature of different progenitors on Type Ia supernova events and tried to observe those stellar systems before the explosion, eventually coming up with contradictory answers. This means that our understanding of Type Ia supernova is not satisfactory, which affects every branch of science where Type Ia supernova play a central role, from cosmology to the chemical enrichment of our Galaxy.
What Causes Type Ia Supernovae Explosions?
It is known that about half of low and intermediate mass stars (M < 8 solar masses) in the Solar Neighborhood have at least one companion. At the end of their evolution, these stars lose their whole envelope, leaving their bare compact carbon-oxygen core, where no nuclear reactions take place, and the whole structure is sustained by the degeneracy pressure of the electons. This object is known as white dwarf. If a white-dwarf is in a binary system, it can accrete matter from a companion, eventually triggering a Type Ia supernova explosion. Two main scenarios are suggested: the single-degenerate scenario and the double-degenerate scenario (see Figure 1).
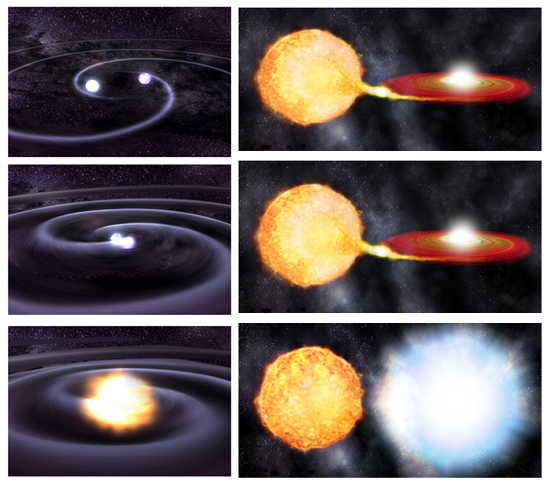
In the single-degenerate scenario, a white-dwarf accretes mass from the envelope of a less evolved stellar companion. When the object approaches the critical Chandrasekhar mass (the maximum mass that can be sustained by electron degeneracy, around 1.39 solar masses for carbon-oxygen white-dwarfs), the whole structure collapses, making the central tempertature and density to grow exponentially. Because of this, carbon atomic nuclei start nuclear fusion chains, igniting explosively and causing… a Type Ia Supernova explosion. An exception can occur when the accreted matter is mainly helium, which would trigger a detonation before the Chandrasekhar mass is reached (an event called Sub-Chandrasekhar Type Ia Supernova). In the double-degenerate scenario, the companion is another WD. The two stars may shrink their orbit, losing potential energy, thereby emitting gravitational waves, until they merge, triggering the supernova explosion.
Measuring distances of the most far away galaxies
Due to their characteristic lightcurve, Type Ia are routinely being used as standard candles for distance measurements of galaxies, which provided evidence for the accelerated expansion of the universe (Nobel Prize Physics 2011). For this reason, Type Ia supernovae are a fantastic tool to investigate the Universe! This is not because Type Ia supernovae peak at the same luminosity, we just saw how they can originate from a variety of scenarios, which is reflected in their lightcurve. But what astronomers realized in 1990s, is that the more luminous a Type Ia Supernova is, the slower it will decrease in luminosity. In other words, even if a Type Ia explosion is not caught at its peak, the evolution of its luminosity even weeks after the explosion can tell us very precisely how bright the explosion was at its peak. With this relation in mind (known as “Philips relation”), astronomers only have to compare Type Ia supernovae from galaxies with known distances with others from galaxies whose distance was unknown. If their luminosity decreases at the same speed, then this means their peak absolute luminosity is also the same. At this point, astronomers only have to compare their different apparent luminosity, to deduce the the distance even of the most far away galaxies we can observe.

Cosmic iron factories!
The key role of Type Ia supernovae in Astronomy goes even beyond simply being distance indicators. Indeed, they are essential contributors to the chemical enrichment of our Galaxy. It is known that about two thirds of the iron content in the Solar System has been forged in Type Ia supernova explosions, which took place billions of years before the formation of the Sun. Those supernovae enriched the pristine Solar Nebula with freshly newly formed chemical elements, above all A LOT of iron (each type Ia supernova event produces on average 0.6 solar masses of iron, that is 200 thousands Earth masses!!), together with high abundances of manganese, silicon and sulfur. Look around you: chances are that the atoms of any object made of iron you may see were forged billions of years ago, within one of these majestic explosions… including the iron atoms present in our own blood! It is really true, we are all made of stars.
Umberto Battino
Cover picture: Hubble Space Telescope-Image of Supernova 1994D (SN1994D) in galaxy NGC 4526, visible on the lower left. Credits: NASA/ESA, The Hubble Key Project Team and The High-Z Supernova Search Team
References:
- Moll et al., ApJ, Volume 785, Issue 2, article id. 105, pp. (2014)
- Phillips, M. M.; ApJ Letters v.413, p.L105 (1993)
- Perlmutter, S. et al., Nature, Volume 391, Issue 6662, pp. 51-54 (1998)
- Riess, A. G. et al., The Astronomical Journal, Volume 116, Issue 3, pp. 1009-1038 (1998)
- Travaglio et al. 2011, The Astrophysical Journal, 739:93 (19pp)